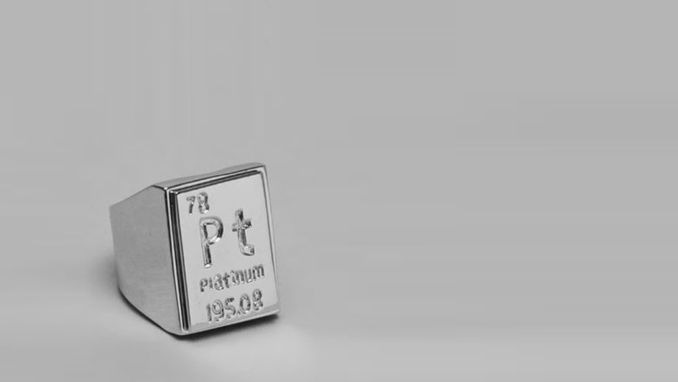
Here we are again, off on our elementary excursions. As I have mentioned before, I hope to ensure that at least one element is included from each of the eighteen Groups of the Periodic Table (a.k.a. the Periodic Table of the Elements).
So far, we’ve had a canter through the stories of elements from eight of these:
- Group 1: Hydrogen, and Sodium
- Group 2: Calcium
- Group 8: Iron
- Group 11: Copper
- Group 12: Mercury
- Group 14: Carbon
- Group 16: Oxygen, and Sulphur
- Group 17: Bromine
This time, we’re going to delve into the delights which an element near the bottom of Group 10 has to offer. This is the story of one of the precious metals (metals like gold and silver, which are relatively rare, hence have a high value). This is platinum’s party piece.
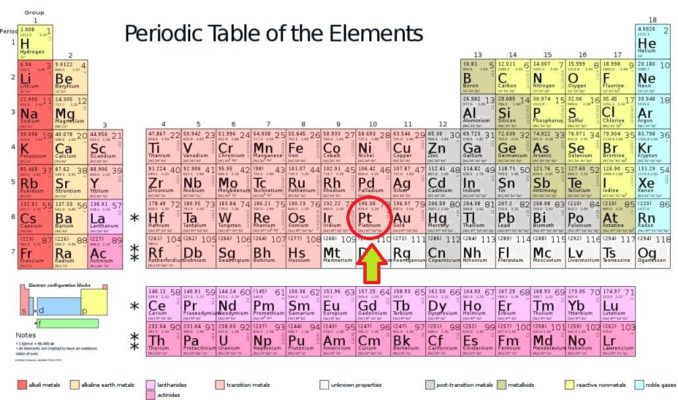
2012rc, licensed under CC BY 3.0
Platinum, which has been assigned the symbol Pt, is the 78th element of the periodic table. It is the heaviest of the stable elements in Group 10, and it is also amongst the densest of all the elements, with a density of 21.45 g/cm3 (only osmium and iridium are beat it for density). This means that a six-inch cube of platinum would weigh around 165 pounds, a little under twelve stone.
Pure platinum is a relatively soft metal so can, just like pure elemental gold, be easily damaged, thus is usually used in alloy form. However, this softness means it is also highly malleable (so is easily shaped or formed by hammering). It can be beaten into very thin sheets, only a hundred atoms thick. It is also extremely ductile (easy to draw or pull into wire). In fact, it is the most ductile of pure metals, so just a single gram of platinum can be stretched into a wire over 2 kilometres in length.
Our element is also one of the least reactive metals. Even at high temperatures it is extremely resistant to corrosion, indeed, even better here than gold. As is expected of a metal, platinum has good electrical and thermal conductivity, thought it falls far short of silver and gold in this respect.
Very similar to copper (which we have explored in a previous article) platinum exhibits a face-centred cubic crystal structure and has no known allotropes.
When it comes to isotopes, platinum has five stable ones. Of these, 192Pt, and 198Pt are not very abundant, but 194Pt, 195Pt, and 196Pt, are much more common, making up the bulk of native platinum. Out of these three, 195Pt is the key isotope, being just about the most abundant. Platinum also has one naturally occurring radioisotope (190Pt). This has a very long half-life (around six hundred and fifty billion years) so could almost be regarded as stable. Additionally, it has well over thirty synthetic (artificial) radioisotopes.
Some of platinum’s isotopes have found a number of uses. To test nuclear models, 196Pt is used. Research into dipole strength employs 194Pt, and 196Pt. Some platinum isotopes are used as precursors for other isotopes used for medicinal purposes, often linked to cancer diagnosis and therapy: 195Pt à 195mPt, 198Pt à 199Au, and 194Pt à 195Hg. After more than forty years, platinum drugs (e.g. cisplatin, cis-diamminedichloroplatinum(II), first approved for use in 1978), are still among the most widely used anticancer drugs.
The name assigned to our element has rather interesting origins. When the 15th century Spanish conquistadores, in the hunt for yet more gold and the fabled El Dorado, ‘discovered’ the platinum which had been in use for centuries in Ecuador and Colombia, they were decidedly unimpressed. They found platinum nuggets (and ores) amidst the gold they collected, but regarded it as inferior, an impurity to be discarded, a nuisance since it was so difficult to work (its melting point is a colossal 1,770° C). There are even reports that the Spanish threw this worthless metal back into rivers in Ecuador to ‘ripen’. Their focus was perhaps a little blinkered, fixated on the gold and silver they knew was there to be acquired. Thus, the name we use today comes from the Spanish pejorative ‘platina del Pinto’, meaning ’little silver of the Pinto River’, though they also described it as oro blanco, or juan blanco.
Platinum’s group, the Group 10 elements (all of which are metals) haven’t been assigned a nice evocative ‘trivial’ name. Sadly, it has this in common with most of the groups which fall into the smart salmon pink section of the table, the transition metals, are sometimes known as the d-block elements, which we’ve met before. Since the elements in this group are attractive metals, silvery-white and lustrous, if I were in charge, I might give them a name something like τα όμορφα, ‘the beautiful ones’. Platinum shares Group 10 with Nickel (Ni), Palladium (Pd), and Darmstadtium (Ds). Let’s take a quick look at these.
A synthetic element, and an extremely radioactive one, Darmstadtium (which was named after the city of Darmstadt, Germany, where it was synthesised in 1994) is still by and large a mystery. To date, fifteen isotopes have been reported for this element, but they have very short half-lives so don’t hang about long. All of the isotopes are extremely unstable, and the most ‘stable’ of them, well, the one which decays least rapidly, 281Ds, has a half-life of only about 12.7 seconds. This means that no properties for either the element or its compounds have been measured. Well, this and the small matter of it being screamingly expensive to synthesise—when it was first observed, in order to fuse lead-208 with nickel-62, scientists had bombarded a lead target with more than a billion billion nickel ions for an entire week. This marathon effort yielded precisely one atom of darmstadtium-269 (269Ds). In theory, if it were possible to keep it around long enough to check, darmstadtium ‘should’ be a very dense and heavy solid, and ‘should’ be a ‘noble’ metal, one which is extremely corrosion resistant.
Palladium (Pd), which is classed as a precious metal, is another pretty rare element (like platinum, it is around thirty times rarer than gold). However, at least this element is a natural one. It has been found in native form (albeit alloyed with a little platinum and iridium) in Brazil and Colombia. But most of the world’s palladium comes from the Ural Mountains in Russia and the Transvaal in South Africa, typically in the form of sulphide minerals. Most of it is extracted commercially as a by-product of the mining and refining of other metals, primarily nickel and our element platinum. That said, the massive industrial and commercial demand for palladium outstrips supply, driving up the prices. It is used, in lesser amounts, in dentistry, electronics, and, of course, in jewellery. But its chief use is as a crucial component in catalytic converters. Because it is such a valuable element, this has led to cars being targeted to ‘harvest’ this and other valuable components—in the five years between 2017 and 2021, over fifty thousand two hundred thefts of catalytic converters were reported to plod. Oh, and only c.1% of these cases resulted in someone being charged.
Nickel (Ni) is the poor relation of the group, as it is not a precious metal. That said, it is one of only four elements which are ferromagnetic at close to room temperature, and it has found a large number of applications. So, it’s a valuable element, even if it isn’t ‘precious’ about it.
Before we move on to look at platinum in more detail, it’s worth saying that this element, together with palladium, rhodium, ruthenium, iridium, and osmium form a sub-group of elements referred to as the platinoids, or the platinum group metals (PGMs). These all cluster closely together on the Periodic Table (see below), are all transition metals, and exhibit quite similar chemical properties. Furthermore, the platinoids all tend to be found in the same mineral deposits.
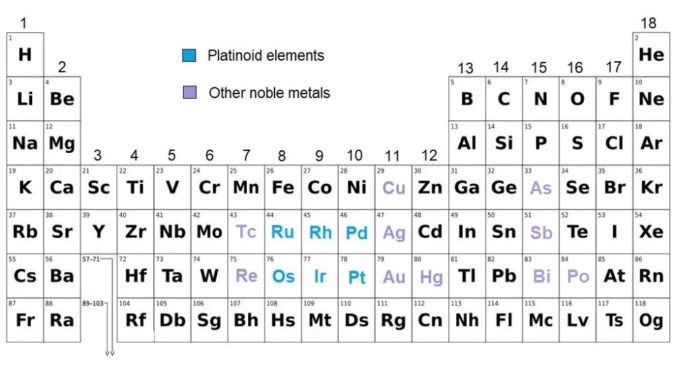
Adapted from László Németh, Public Domain
Additionally, the platnoids are classed as ‘noble’ metals, others of which are also found nearby on the Table. The noble metals, including the platnoids, are typically corrosion resistant and can usually be found in their native (elemental) form in nature. That said, which elements are included as ‘noble’ depends on who you ask. The table above includes every metal which has, correctly or not, been given this categorisation.
The platinoids also overlap quite heavily with the ‘precious’ metals. These, of course, include gold, silver, and platinum, as you might expect from a visit to a jeweller, but also iridium, osmium, palladium, rhenium, rhodium, and ruthenium.

Adapted from Tomihahndorf, licensed under CC BY-SA 3.0
In prestige terms, we consider platinum to be the finest of the precious metals. We still assign the term ‘platinum’, denoting the best of the best, to things like credit cards, fine whisky, blondes (e.g. actresses Jean Harlow, Jayne Mansfield, Diana Dors, and Marilyn Monroe), and music discs—records, in old git parlance.
We have already seen, from the origins of its name, that platinum was known and used by pre-Columbian Andean civilisations of South America. They ‘mined’ the metal which had formed when minerals eroded from their original location and were carried by water to somewhere else (this is stream bed, or alluvial mining).
Our metal, along with precious metals such as gold and silver, was certainly being worked by the Tumaco-La Tolita culture from northwest Ecuador/southwest Colombia, between 600 BC and 200 AD. They used the metals to produce artistic objects such as masks (often combining gold and platinum), items of adornment like nose rings and other jewellery, and small tools, such as needles, tweezers, awls, and fishhooks.
However, there is some evidence to suggest that the first platinum to be worked may pre-date the Tumaco-La Tolita by around two hundred years. The Chorrera culture, who established themselves on Ecuador’s coastal plains about 1,300 BC certainly made use of the local copper, silver, and gold, and it is thought that platinum may have been added to their repertoire around 800 BC. The Chorrera would almost certainly have been able to distinguish platinum from silver. In his ‘Analysis of ancient metals‘, the chemist and metallurgist Earle R. Caley points out that:
“Ancient objects composed of platinum or platinum alloys are easily distinguished from ancient silver objects by their freedom from corrosion products and their much higher specific gravity.”
The same could be said for these elements found as nuggets of native metals. The use of precious metals continued in this region, certainly up to the eruption of the Pululahua volcano in 467 BC. It also endured afterwards in the areas unaffected by the volcanic disruption. This included the north of Ecuador, towards the border with what is now Colombia—Tumaco-La Tolita territory. This expertise in metallurgy remained the state of affairs throughout the remainder of Pre-Columbian history, including the period after this territory was invaded by the Inca in the mid-1400s.
Precise dates for the pre-Columbian objects which remain intact (i.e. not melted down by the conquistadores) are generally not easy to come by. This is, in part, because of the inconsistent nature of archaeology in the region until more recent times. However, this in turn was not helped by the confusion created by hundreds of years of ‘gold fever’. This fervent avarice followed Spanish, and eventually other European colonisation of the Americas after Columbus’s discovery of the South American continent (he made landfall on the coast of modern-day Venezuela in 1498, on his third voyage).
By 1532, when Francisco Pizarro and his conquistadors arrived in this part of Ecuador, much of the region, together with its rich resources, was ripe for the picking. The Spanish had reached the area right on the heels of a period of bitter civil war for the Inca interlopers, who had themselves not long settled in the region. The Incan victor in this struggle, Atahualpa, and his people were exhausted after defeating his rebellious brother Huáscar. Thus, the Spanish conquistadores faced less resistance than they might otherwise have done, advancing rapidly through the terrain, particularly as they came with horses, armour, and firearms.
Nevertheless, the oldest (yet) verifiably dated material evidence of the metal’s use comes from ancient Egypt, and dates to the 7th century BC. A number of small items of jewellery from this period and earlier are known to contain platinum but this may not prove intentional use (platinum naturally occurs in ores imported from Nubia). But platinum does appear to have been used deliberately on a small box or casket found at Thebes in Upper Egypt, now in the possession of the Louvre Museum, Department of Egyptian Antiquities.
This small box, just five and a half inches tall, is decorated with hieroglyphs in both gold and ‘silvery’ metal, with the name Shepenupet II. She was a 25th Dynasty princess who was high priestess in the temple of the god Amun around 700 – 650 BC. Analysis showed that some of the silvery metal was indeed silver, but other sections were native platinum (albeit mixed with small quantities of gold and iridium).
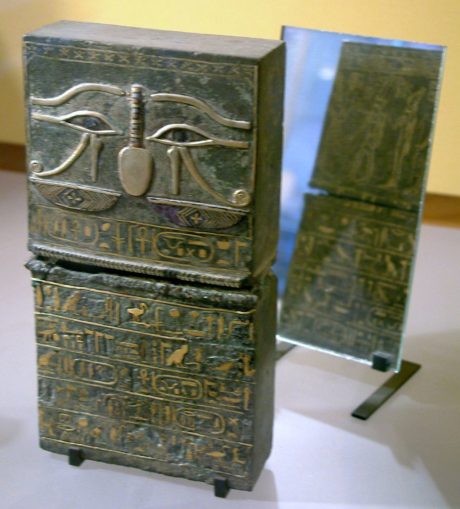
Guillaume Blanchard, licensed under CC BY-SA 3.0
Despite these Egyptian finds, the man usually credited with the ‘discovery’ of platinum takes us back to the Spanish conquest of South America. He is the Sevillano man, Antonio de Ulloa—in full, the magnificently named Antonio de Ulloa y de la Torre-Giralt.
A bright, intelligent young man, at the age of just nineteen he’d already racked up six years of exploratory experience and had risen through the naval ranks to lieutenant. Ulloa was appointed to accompany the 1735 Expédition géodésique française en Équateur (French Geodesic Mission) led by Pierre Bouguer, who travelled to what we now know as Ecuador. During this eight-year-long mission Ulloa recorded masses of information in a series of reports about astronomy (the primary purpose of the mission was to measure the arc of the meridian near Quito), but also about the region’s inhabitants and natural history.
In one of Ulloa’s reports, he described a metal that he called platina. He describes this as a product of mining with “such resistance, that it is not easy to break or cut with the force of blows on a steel anvil” and one which “resists calcination”. He also remarked that “in the district of Choco are many mines of lavadero or wash gold … several mines have been abandoned on account of the platina.” As you can see, the Spanish were not impressed with this nuisance metal.
Although it may be that Ulloa retains the credit for the ‘discovery’ of our element, that pesky ‘platina’ had been noticed many years before. A manuscript in the Archivo Nacional de Colombia, dated 1726, unequivocally indicates that it had been recognised by the Spanish prior to this. The ‘Bogotá Manuscript’ written by José Sánchez de la Torre y Armas refers to a cost analysis for refining a batch of gold which contained platinum. This does indeed push the date back, but to be entirely accurate, I’d say the true discovers were most likely the Chorrera peoples of Ecuador.
In any case, after eventually returning to Spain Ulloa published his Relación histórica del viaje a la América Meridional, in 1748. His return trip wasn’t without its perils—Ulloa was arrested offshore and held as a prisoner of war in London when his ship, the Deliverance, was captured by the British Royal Navy. Amazingly, during his imprisonment, his scientific expertise had been recognised from the reports he carried, and so greatly valued that, in 1746, Ulloa was elected as a Fellow of the Royal Society of London!
Interestingly, around the same period, there was already some burgeoning scientific curiosity in England about our element. Bear in mind that this was in the midst of the ‘War of Jenkins’ Ear’ (1739 – 48), part of an ongoing conflict over access to resources and markets in Spanish America, the Anglo-Spanish Wars, which rolled on for centuries between the British and Spanish Empires. Getting hold of samples of this intriguing new material was not exactly straightforward.
Nonetheless, it wasn’t completely out of the question. In 1741, the Wolverhampton ironmaster Charles Wood (who had been instrumental in developing a novel ironmaking process, ‘potting and stamping’, to produce wrought iron from pig iron) headed to Jamaica. There, he came into contact with some of the unusual metal samples (platina) that had been smuggled out of Cartagena de Indias, the port of Nueva Granada (in present-day Colombia). Being “a skilful and inquisitive metallurgist”, Wood, of course, set to and conducted a series of experiments on this odd metal.
His interest was thoroughly piqued, but his resources were somewhat lacking, so Wood sent samples to his brother-in-law, William Brownrigg, back in Britain. Brownrigg was a brilliant but rather self-effacing man. A doctor, and scientist, based in Whitehaven, Cumberland, he had become a Fellow of the Royal Society of London in 1742. Brownrigg continued studying the new metal, repeating some of Wood’s experiments and expanding upon these. He eventually gave his findings (along with samples of platina) to William Watson, another member of the Royal Society, who presented them to the Society on Brownrigg’s behalf. A copy of this communication can be seen HERE.
It is interesting that, in a time when their respective countries were sworn enemies, the amity of science took precedence. Watson used the term ‘platina’ for the metal in his presentation to the Royal Society, giving credit to the Spaniard, Ulloa, saying:
“This substance has not been mentioned by any author I know, with the exception of our esteemed Antonio de Ulloa”.
Watson’s (and Brownrigg’s) paper meant the news of ‘platina’ was truly out in Europe. This created considerable interest in scientific circles. Work on purifying and refining this metal and establishing possible applications for it abounded in countries across Europe.
By now, this new and rare metal, platinum, had also come to the attention of the extensive brotherhood of alchemists. It rapidly became a valued ingredient in the cockamamie concoctions they formulated in their quest to transmute base metals, very often with lead as a starting point, into gold.
Since this metal exhibited a set of quite recognisable properties, some alchemists reasoned that it must be an ‘amalgam’ of gold and silver. Thus, although it was given a glyph of its own for its alchemical symbol, as we can see below, this combined the sun symbol (denoting gold) and the moon symbol (for silver).

SharpieType301, 2023
There was a small snag though. No-one could figure out a way to melt this extraordinary metal. Although a ‘partial agglomeration’ was achieved, using platinum in a powdered form, and it was possible to melt platinum with copper, or arsenic, this did not provide the alchemists with the homogeneous, pure molten metal they desired.
Even subjecting it to the rays of the famed ‘Tschirnhaus lens’ or ‘Burning Glass’ (which we have seen in previous articles), failed to melt our recalcitrant element. It took until the 1780s before our old friend Antoine-Laurent de Lavoisier managed to completely melt minute quantities of the metal. He had first attempted this, using a colossal 47¹/₄ inch diameter ‘Burning Glass’. Nope, that showed a little promise, but didn’t work, so he tried again, and again, changing the techniques he tried until he finally succeeded in his endeavours.
In June 1782, Lavoisier demonstrated his tremendous discovery to Benjamin Franklin. He wrote to his good friend Joseph Priestley describing how Lavoisier had accomplished this feat:
“Yesterday the Count du Nord was at the Academy of Sciences, when sundry Experiments were exhibited for his Entertainment; among them, one by M. Lavoisier, to show that the strongest Fire we yet know, is made in a Charcoal blown on with dephlogisticated air. In a Heat so produced, he melted Platina presently, the Fire being much more powerful than that of the strongest burning mirror.”
We’ve discussed ‘dephlogisticated air’ in a previous article. This is the gas oxygen, which Priestley himself had isolated in pure, elemental form in 1774.
But despite the considerable interest in the new metal, throughout this time the Spanish controlled the source of ‘platina’. Perhaps unsurprisingly then, in 1786, it was a Spaniards, brothers Fausto de Elhúyar and Juan José Elhúyar Lubize, together with their French collaborator, Pierre François Chabaneau, who devised a process to refine platinum. This allowed them to remove traces of impurities such as gold, iron, mercury, lead, and copper from their samples.
This produced a malleable metal, but the process, which involved powder metallurgy and extreme heat, was kept a close secret on the instruction of the King Carlos III of Spain. Fausto was appointed General Director of Mines in Mexico, so his interest in platinum fell away as he left Spain and his focus switched to silver. Juan José also headed to South America, studying minerals from New Granada, but also from Ecuador and Peru.
Back in Spain, the grateful King provided Chabaneau with a laboratory in Madrid. It wasn’t all plain sailing though. Although the common impurities could be removed, the quality of the refined metal was frustratingly inconsistent. What no-one knew at the time was that other elements were present in the mix. These impurities, which had not been identified at that point, were other platinoid elements: iridium, osmium, palladium, rhodium, and ruthenium.
Chabaneau’s novel process remained a secret until 1914. In part, this was the to fulfil the monarch’s wish. However, canny Chabaneau was also well aware that the challenges around of working with this metal gave it a particular cachet, adding significant value to any objects which were made with it. Hence, he and his colleagues began to produce platinum ingots for use by craftsmen. Thus began Spain’s ‘Platinum Age’.
He took some of this metal to France, where he visited Marc-Etienne Janety, a talented silversmith, and the Royal Goldsmith to King Louis XVI. The French King had declared platinum to be the “only metal fit for kings”. True to his word to the Spanish monarch, Chabaneau did not disclose his refining secrets, but Janety devised a technique of his own.
He used ’white arsenic’ in a very dangerous process which he devised to remove base metals from native platinum. This was a multi-step process, which involved first treating the native metal with three or four times its weight of ’white arsenic’ (arsenious acid, H3AsO3) and a few ounces of ’salt of tartar’ (potassium carbonate, K2CO3). That was effective, but the next problem was to remove the arsenic. Despite all of the evident obstacles, this allowed Janety to produce platinum in large amounts in a pure form, which was marvellously malleable.
Lavoisier reported to the Académie des Sciences in 1790. In his paper ‘Observations sur la platine‘ for the ‘Annales De Chimie’, he described Janety’s process for refining platinum. He was so excited that he emphasised that there was now “no limit to the type of vessel that could be produced in platinum”.
Lowering of platinum’s melting point had also allowed the metal to be cast. Using his own complex and hazardous method to refine the metal, Janety created a number of beautiful ornamental platinum objects. These include jewellery, snuffboxes, watch-chains, coffee-pots, etc. Of these, only one survives today, the charming cobalt glass-lined sugar bowl you see below.
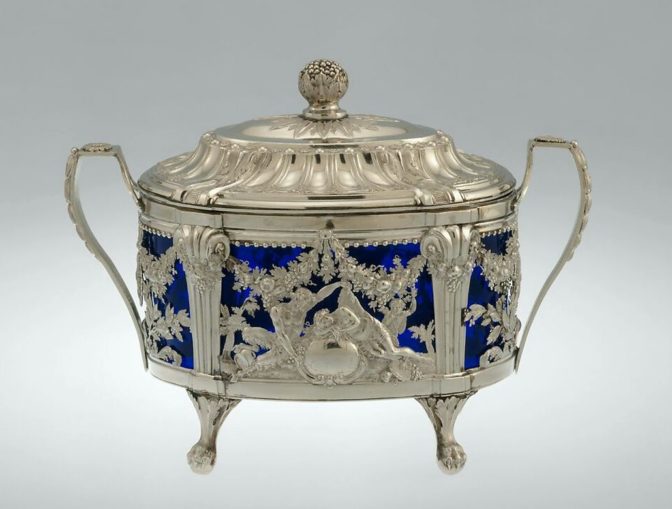
The Metropolitan Museum of Art, Public Domain
Sadly, this exciting aesthetic era was not to last for long. The work which had been carried out in France came to an end because of the French Revolution (when even renowned scientists like Lavoisier fell under suspicion and were, in fact, executed). Furthermore, with France and Spain no longer allies, the Spanish Empire crumbled in the early 1800s under the onslaught of the Napoleonic wars.
Perhaps inspired by the massive changes in European cultures, the South American countries took their chance to shake off the hated colonial rule. Indeed, most of Latin America achieved its independence by 1825. For some time, this meant that supplies of our metal and its ores from South America more or less dried up. For the next hundred years or so, research into the metal still continued, around Europe, but on a greatly reduced scale.
However, by the late 1800s, high-temperature blowtorches and new methods to shape the metal were introduced to the jewellery trade. Alternative sources of platinum were also discovered in Canada, Southern Africa, and in Russia (where it was known as the ‘new Siberian metal’). This permitted the manufacture of platinum jewellery to begin to flourish. So much so, in fact, that platinum mania spread across the whole of Europe and Russia.
Royalty, whether they be kings, queens, tsars, or maharajas, enveloped themselves in jewellery made from this high-status metal. King Carlos IV of Spain even commissioned a platinum ‘room’ (photographs of the gorgeous Gabinete de platino can be seen via the link to a Spanish publication). This is an exquisitely decorated barrel-vaulted room, enhanced with rich hardwoods inlaid with platinum plate, built at the Real Casa del Labrador at the Royal Palace of Aranjuez. By the beginning of the 20th century, platinum was firmly established as a status symbol, and continued to be during the Edwardian Period.
But enough of the history, for now at least. Let’s take a look at how we now source this precious metal, and at what platinum is used for.
The top five producers of platinum at the moment are South Africa (who mine c. 120 tonnes per year and have the largest reserves, actually more than 90% of the world’s platinoid reserves), Russia (at c. 23 tonnes p.a.), Zimbabwe (c. 13 tonnes p.a.), Canada (c. 9 tonnes p.a.), and the United States, who are the main importers and users despite mining c. 3.9 tonnes every year.
It is not a common metal. In 2022, mine production of platinum worldwide was about 190 tonnes, a.k.a. metric tons (just under 200 proper tons). Contrast this with the same year for gold, where 3,100 tonnes was mined (almost 3,420 tons in old money), around sixteen times as much. This amount is predicted to rise in 2023, as supply still outstrips demand.
The differential speaks to platinum’s rarity, but also to the ease (or lack of it) with which the pure metal can be both mined and extracted from its ores. Most platinum comes from a mineral called cooperite, which is primarily platinum sulphide. Most of the mining for platinum ore occurs deep underground, so it’s a big-ticket ore to extract.
As a little aside, it is believed that one day, it ‘might’ become feasible to ‘mine’ the platinum we need from sources in space (actually that’s a fair sized ‘might’, as this is still very much pie in the sky right now). While it may seem just a sci-fi dream, it has been determined that many Near-Earth Objects (NEOs), a.k.a. asteroids, are rich in heavy elements, like platinum and its fellow platinoids. In 2015, a major ‘space law’ was put in place by Obama (may his name be blessed!) to legally exploit the minerals in such extraterrestrial bodies. While the miners would have no rights of ownership over the hunk of asteroid rock they exploit, they will own anything they can mine from it. There’s already a Californian start-up, AstroForge, with plans to give it a go. Given OceanGate’s recent exploits, I will leave you to draw your own conclusions.
As well as mining to obtain the ores, refining, the process required to produce pure platinum from its ores, is another expensive, time-consuming, and painstaking process. Depending on the particular characteristics of the ore, it can take anything from eight weeks to six months to process a batch of ore, using the Kell hydrometallurgical process. Furthermore, you may need to process a helluva lot of ore, up to 11 tonnes (er, c. 12 tons), to yield a single troy ounce of platinum.
This goes some way to explaining the statement made by Jenny Luker, president of Platinum Guild International USA (PGI), a marketing organization for the platinum jewellery industry. She remarked that if:
“…all the platinum ever mined were melted and poured into an Olympic-sized pool, the platinum would barely reach your ankles. Gold, however, would fill three pools.”
No wonder there is also now growing interest in the recovery and extraction of platinum from spent catalysts (which we will discuss in more detail later).
As a measure of its importance, platinum, together with its alloys, was viewed by the United States government as an important strategic defence metal (critical to U.S. national security and the economy) during World War II. At that time, its use in jewellery making was prohibited.
Platinum endures amongst the materials of ‘strategic’ importance listed under United States federal law, as part of the ‘Strategic and Critical Materials Stock Piling Act of 1939’, which remains in effect to this day. The UK has platinum listed in a similar ‘UK Critical Minerals Strategy’, updated most recently in March 2023, for similar reasons.
OK, so it’s important. But who uses this stuff, and why?
Jewellery, although important in platinum’s story, is actually only a minor application in the history of our metal. Almost half of the platinum mined is used to produce catalytic converters (vehicle exhaust emission control devices). Catalytic converters started to be fitted to cars (but also vehicles such as buses, and lorries) in the mid-1970s when US regulations on air quality changed, not long after Tricky Dicky established the US Environmental Protection Agency (EPA). European countries soon followed suit. The ‘cats’ are the ugly lump, partway down your nice, streamlined exhaust system, close to the engine.
The presence of platinum, and other precious metals in the ‘cats’ fitted to cars brings with it a bit of a problem though. Once a catalytic converter fails, the precious metals inside (e.g. platinum, palladium, and rhodium) can be extracted and recycled. Great, you might think.
The problem comes because the ‘cat’ doesn’t have to fail to be able to gain access to the metals. As we saw earlier, when we took a brief look at palladium, the increasing monetary value of these rare and costly metals has encouraged thieving toerags to steal catalytic converters at an increasingly alarming rate.
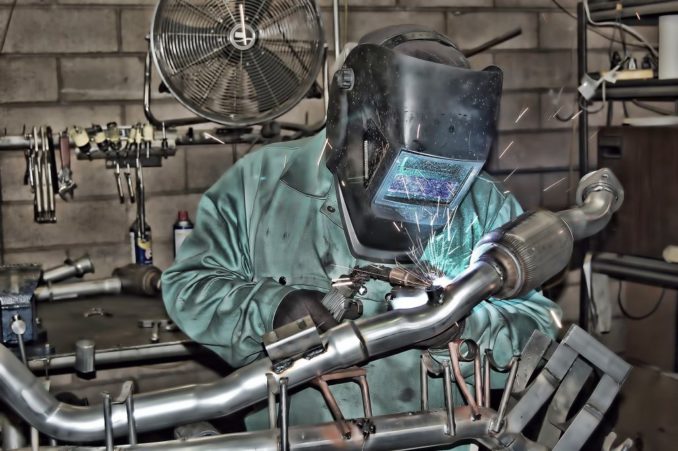
Chris Yarzab, licensed under CC BY 2.0
This isn’t the only use for platinum in your car though. The air bags which are fitted as a safety feature are often coated with platinum-cured silicone. This allows them to remain folded for extended periods without deteriorating, so they’ll still inflate to function as required, when required. In addition, the air bags have an initiator sensor. This is a ‘resistance wire’, thin platinum wire coated with a small quantity of an agent that causes the bag to inflate.
Platinum-based sensors are also used in the carbon monoxide (CO) detectors you may have fitted in your home, and in ‘biosensors’, including the breathalysers used to measure blood alcohol content (car safety again!) or blood glucose, to help diabetics monitor their condition.
Other medicinal uses for our element exist too. We’ve already discussed chemotherapy medication. However, platinum’s excellent corrosion resistance, and good mechanical resistance, and biocompatibility (enabling it to remain in contact with the body without generating any adverse effects), make it ideal for use in implants. The ‘stents’, mesh tubes now used to hold arteries open improving blood flow are increasingly platinum based. Pacemakers, implantable defibrillators, dental implants, artificial joints, plates, rods and pins, some catheters, and other medical devices may also be constructed, wholly or in part, from platinum or its alloys (platinum-tungsten, platinum-nickel, and platinum-iridium alloys are commonly used).
Platinum’s catalytic properties mean that it is used to make chemicals like benzene, and also silicones (used, for example, in sealants, insulation for electrical wires, lubricants, and kitchen implements, oh, and car air bags). It is also used in the first stage of the Ostwald process, in which ammonia is converted to nitric acid via a platinum catalyst. The main industrial use for the nitric acid goes into the production of agricultural fertilisers. Other uses are found in the explosives industry, to produce synthetic fibres (e.g. nylon, polyurethane and other common plastics), some biodegradable materials, and produce specialty organic compounds.
The fact that our metal stands up well under high temperatures and is highly resistant to chemical attack makes it helpful in other fields too. Turbine blades, both in aircraft and power turbines (where temperatures can reach upwards of 2,000°C), are plated with platinum. This enhances their in-service lifespan considerably.
Another important use for platinum is in glass manufacturing, and once again this makes use of the metal’s high melting point. Restraining and directing molten glass needs specialist tools. They need to be able to withstand the elevated temperatures of the glass in its molten state (between 1,400 and 1,600°C). It is also helpful if they can circumvent corrosion and don’t react with components of the glass mix. Platinum is the ideal material here.
For example, fibreglass, whether that fluffy, unstructured, orangey-pink insulation laid down in the attic, or the stiff whitish sheets of woven glass strands that you use to mend the rusted bits on your rattletrap car (or was that just me) is made from extremely fine filaments of glass. But how are these formed?

NoiseD, licensed under CC BY-SA 3.0
To produce fibreglass, a glass (usually a type called E-glass, alumino-borosilicate glass) is heated until liquid, then the molten material is forced through superfine holes in a mesh sieve or grid, which is called a ‘bushing’. This grid is made from hardened grade of platinum, often alloyed with rhodium. The process, whereby the solidifying strands are then pulled through a vat of a binder (usually a resin) to hold them into a usable form is known by the inelegant name, ‘pultrusion’.
You’ll find platinum (albeit in small quantities) in the device on which you are reading this article. It’s in your computer’s guts, in your computer screen, and in mobile phones (there will typically be ≤ 0.001g of platinum in your phone). It’s in hard drives (part of the platters of the old school type, not the newfangled SSDs), in sensors, integrated circuits, Schottky contacts, conductors, capacitors, and in the crucibles in which crystals are produced for liquid crystal displays (LCDs).
We’ve considered the use of platinum in high-status jewellery, but even into more modern times, when we think about our metal, we still immediately associate platinum with good quality (and an elevated price tag) jewellery. This gives me the perfect opportunity to be girly for a moment and drool a little. Cartier… Bulgari… Chopard… Van Cleef & Arpels… Tiffany & Co. of New York City…
The Wade Necklace seen below was made for Ellen Garretson Wade (a prominent Cleveland philanthropist and public benefactor), c.1900. The delightfully decadent platinum, diamond, and sapphire bracelets are a little later, and were designed for Tiffany in the 1920s.
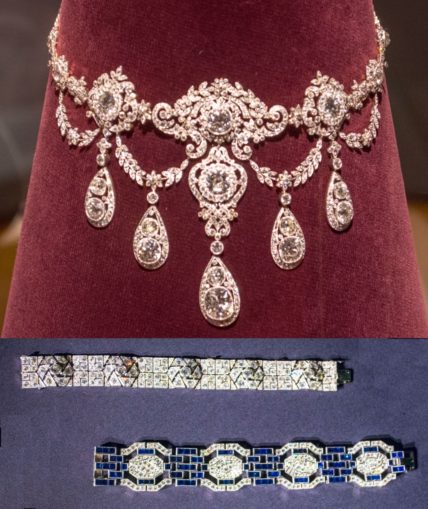
Tim Evanson, licensed under CC BY-SA 2.0
Would I wear these beautiful pieces? Actually no, but I would love to see them and appreciate the exquisite craftmanship of these objects in all their glory.
Another objet d’art, or rather series of objets, which make liberal use of platinum, and that I would give my eye teeth to see are the bejewelled Easter Eggs commissioned by Tsar Alexander III, and his son and successor Tsar Nicholas II, as Easter gifts for their wives and mothers. These men, at the head of the Imperial House of Romanov, were successively Emperor of Russia, King of Congress Poland, and Grand Duke of Finland. The Easter gifts they commissioned are the famed eggs from the House of Fabergé, in Saint Petersburg. Fabergé made nearly seventy eggs in total, but the ones best known were the fifty-two ‘Imperial’ eggs, made for the Romanovs.
Peter Carl Fabergé, otherwise known as Peter Karl Gustavovich Faberzhe, is synonymous with opulence. He was considered the finest goldsmith of his time. He, and the House of Fabergé, are worthy of a (very long and detailed) article in their own right.
Fabergé was a strict taskmaster, with exacting standards and a clear view of what was and was not acceptable, and this shows in the exquisite work from his studios. It is rumoured that, when he examined the workmanship of his craftsmen, he had a stamp, and a hammer with him. The piece in question would either receive his stamp of approval (literally) or would be beaten with the hammer to destroy it.
Before Easter in 1885, Tsar Alexander III’s brother, the Grand Duke Vladimir Alexandrovich, suggested that Peter Carl Fabergé create a jewelled egg. This first egg was to be a gift for the Tsar’s beloved wife, the “sweetly pretty” Maria Feodorovna, known before her marriage as Princess Dagmar of Denmark.
The design of this egg was said to have been inspired by an item of jewellery (the ‘Wilhelmina’ egg) held in the collections of the Danish Royal family, and owned by Maria’s aunt, Princess Wilhelmine Marie of Denmark. The egg had originally been made in France, in 1720, for Élisabeth Charlotte d’Orléans, Princess of Commercy.
The Danish egg, which was ivory, not gold, and conceals a ring inside, is still in the Royal Collections. This was a familiar object to Alexander’s Tsarina, who told her husband how she had admired it in her childhood. The Tsar thought a similar egg would be the perfect distraction for her to the unpleasant events of that time (the royal family received frequent death threats). He was right. She loved it!
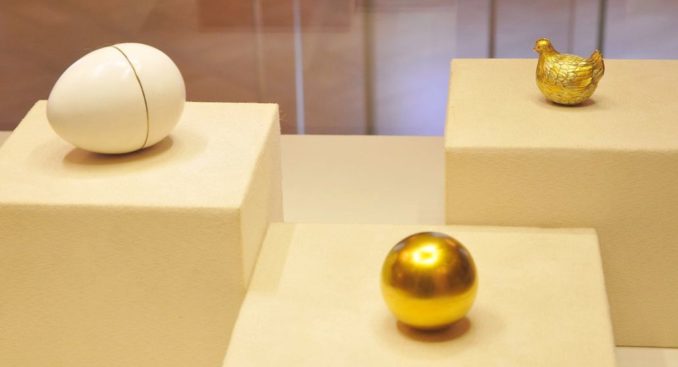
Михаил Овчинников, licensed under CC BY-SA 4.0
The making of Fabergé’s fabulous ‘Hen Egg’ was the beginning of an extraordinary period of work, spanning more than three decades. In all, some fifty-two ‘Imperial’ eggs were produced, of which most have, thankfully, survived.
That said, after the Russian Revolution, the abdication of Tsar Nicholas II and the fall of House of Romanov, and the subsequent execution of the royal family, chaos ensued. Fabergé himself didn’t fare well, as the Bolsheviks nationalised his business and he was forced to flee to Switzerland, where he died a few years later. Since that time the famed Fabergé trademark has exchanged hands many times.
In theory, the Romanov’s Fabergé eggs, including the ‘Hen Egg’, were secure. Vladimir Lenin had ordered that they, and many other treasures from the Imperial palaces, be moved to the safety of the Kremlin Armoury (indeed, nine are still there). Nonetheless, some of the eggs were ‘lost’ (make of that what you will).
Many more must have ended up under State control though, probably housed in the Kremlin, as Joseph Stalin arranged for a number of Fabergé’s eggs to be sold in 1927. His aim was to use these hated symbols of the opulent ‘old ways’ to buttress the new Russian Soviet Federative Socialist Republic by the acquisition of more foreign currency.
Of almost seventy eggs that the House of Fabergé made, fifty-seven are known to exist to this day. The hunt for others continues, but the eggs that remain are scattered in many locations. A good number, fifteen at last count, are in the private ownership of Ukrainian-Russian–Israeli-Cypriot oligarch, Viktor Vekselberg. The nine ‘Imperial’ eggs in his collection are displayed in the Fabergé Museum in Saint Petersburg. They include the ‘Hen Egg’, sadly no longer complete, missing as it is the miniature Imperial crown and ruby egg-pendant which the little hen had originally concealed.
Details of this egg, and others, can be seen through the Fabergé eggs link at the top of the Fabergé Discoveries website. I’ll give you fair warning. Once you click on this website, you won’t be seen for some time. It pulls you into the most magical world of wonderful workmanship and immutable splendour.
I’ll finish this piece with what is, to me, a slightly sad story, and one which is reflective of our modern times. We Brits cling to what we consider ‘proper’ measurements, the pounds, and ounces, amongst other Imperial measurements, a.k.a. the British Imperial System. That said, as an old lab rat, I spent a great deal of my life using the Metric system, and important amongst these measurements was the Kilogram.
The Kilogram’s history goes back to the ‘Age of Enlightenment’ (a.k.a. the ‘Age of Reason’) in the 17th and 18th centuries, and to the French Revolution, which fell towards the end of this period. In 1790, King Louis XVI of France ordered a new system of measurement. This was to be standardised, “for all people, in all places, for all time”. It was hoped that this would help control the uninhibited fraudulence which was common under the existing system which comprised numerous localised systems of weights and measures.
Talleyrand (Charles-Maurice de Talleyrand-Périgord), a.k.a. ‘le diable boiteux’, or ‘the lame devil’, was instrumental in devising this system. It was to be one based on natural units. Talleyrand was a decidedly odd man. A defrocked Bishop, he was, by all accounts, also a bit of a lad, having fathered a couple of dozen illegitimate offspring. However, no matter what his personal life held, a new unit of mass was dutifully created.
This was the ‘grave’, which was renamed, post-French Revolution, as the kilogram. The ‘grave’ was defined as the unit of mass of a cubic decimetre (a litre) of distilled water at 0 °C. Our old friend Lavoisier gets in on the act here as well. He and René Just Haüy confirmed that this (as yet provisional) kilogram equated to 18,841 ‘grains’, a mass measurement traditionally used in apothecaries’ systems.
So far, so good, but it was quickly realised that a ‘standard’ mass based on a pot of water, especially at 0 °C, was just a tad inconvenient. So, in 1793, a one-piece, metallic ‘grave’ or kilogram mass was produced.
It is very likely (as you might guess from the picture below) that this was made of copper, as Thomas Jefferson is known to have requested a 1kg copper cylinder from France, to use as a ‘standard’, so that the metric system could be adopted in the United States. Unfortunately, the ship carrying this was hit by both storms and pirates, so the Americans dodged the metrification bullet a while longer.
In hindsight, copper might not have been the best choice for a ‘standard’ mass either, since it is a reactive metal. It tarnishes readily, reacting with moisture, oxygen, and carbon dioxide in the air. This forms a thin blue-green layer of Cu2CO3(OH)2 (copper (II) carbonate hydroxide), over the surface of the pinky-orange pure metal, affecting its mass.
Then, in 1799, an all-platinum kilogram prototype was made, in fact cast by the jeweller Marc-Etienne Janety who we encountered earlier in this piece. Theoretically speaking, this was much better as platinum is one of the least reactive metals. Still ‘provisional’ at this point, the idea was that this ‘kilogram’ should equal (as closely as was scientifically possible to achieve) the mass of one cubic decimetre of water at 4 °C (the temperature at which water reaches maximum density). It was titled the ‘Kilogramme des Archives’ after the Archives Nationales in Paris where this hunk of metal was housed. This new standard really was ‘the kilogram’, and it prevailed for the next ninety years.

Adapted from National Institute for Standards and Technology, Public Domain
Janety’s kilogram was finally replaced in 1889 by the International Prototype of the Kilogram (IPK), which was made in London ten years previously by Johnson Matthey. This time, instead of pure platinum, the kilogram was made of platinum–iridium alloy. This 90% platinum and 10% iridium (by mass) alloy retained all the chemical stability of pure platinum, but was a harder, more durable material.
The IPK (and six sister copies) was securely stored under a matryoshka-like system of double glass bell jars at the International Bureau of Weights and Measures in Paris. Additional copies were made and stored in a similar fashion at locations in forty-four countries around the world, to act as local standards.
All was good, and the IPK reigned supreme until 2019, with the standards cleaned periodically, following the ‘BIPM cleaning method’ which, as can be seen in the linked paper, is a very rigorously controlled process, but not foolproof. It appears that the mass actually did change a miniscule amount over time.
Now we come to the sad part. The IPK, as is a physical kilogram standard that can be seen and touched, is no more. After 130 years of devoted service, the platinum–iridium ‘kilogram’ standards have been put out to pasture. The new standard is founded, not on a physical object, but in physics alone, based on the Planck constant and defined in terms of an electric current. The kilogram has gone ‘virtual’.
Poor Talleyrand must be rolling in his ‘grave’ (pun intended). Although he is often seen as a bad boy (A traitor? A patriot? …or perhaps both), backing both horses in the French Revolution, and switching saddles as it best suited his personal interests, he had a way with words. Not a thing to do with platinum, but one of his quotes stuck a particular chord, so I will leave you with Charles Maurice de Talleyrand-Périgord.
Adapted from Cris7125, licensed under CC BY-SA 4.0
© SharpieType301 2023